If you're just starting out with Arduino, you might be wondering what projects you can take on to practice your skills. Don’t worry we got you covered. We have created this massive list of 37 beginner friendly Arduino projects for you. This guide offers over 30 beginner-friendly project ideas, complete with code and circuit diagrams, to help you get started. Whether you're interested in home automation, robotics, or simple iot projects, there's something here to spark your creativity and help you learn the Arduino environment. From simple motion sensor projects to more complex systems like automated plant waterers or remote-controlled robots, you’ll find plenty of inspiration here. Each project is designed to build your confidence and expand your understanding of how Arduino works. So, grab your Arduino, pick a project that excites you, and dive in—there’s no better way to start mastering the world of electronics!
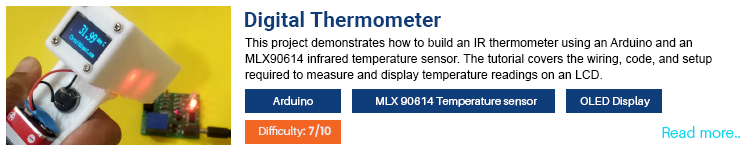
In our daily lives, we use thermometers on various occasions and for various purposes, from medical needs to repair and diagnostic purposes. As you know there are different types of thermometers, and one main classification would be based on how they operate. Some would need to be in contact with the subject to read the temperature. The main issue with these types is that if the contact is it proper the readings would also be inaccurate. Not only that these types of devices are impractical to use during pandemics like COVID. That's where the contactless thermometers come in handy. They offer good accuracy without any of the drawbacks of other types of thermometers. These types of thermometers use infrared sensors to measure infrared radiation from a hot body to accurately calculate the temperature. So, in this project, we will explain how we can make such a non-contact infrared thermometer using an MLX90614 sensor And an Arduino.
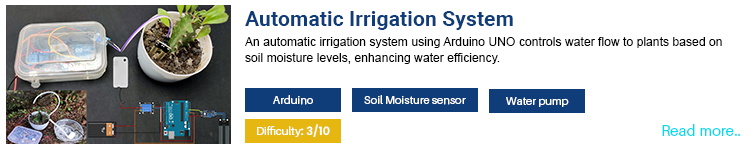
We all like a bit of greenery around, whether it's indoor plants or a garden. They always help us to keep us calm and relaxed. Even though they are low maintenance compared to pets, they need periodic care to keep them alive and fresh. The main concern for plants is soil moisture. It should be at a specific level to keep the plants healthy. Both over watering as well as not watering enough can affect the plant's health. In such situations, we use automatic watering systems to keep the soil moisture at a required level. But the problem is that the commercial solutions are expensive and are not that affordable. That’s why, in this project, we are going to make an automatic irrigation system using an Arduino and a few other cheap and easy-to-find components. We will use a soil moisture sensor to detect the moisture level and then activate a water pump as needed to keep the mixture at the desired level.
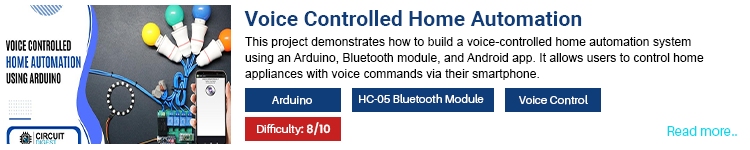
We all know how convenient is to use home automation and voice assistant systems such as Google Home, Apple HomeKit or Amazon Echo. They all offer a convenient and easy way to control our devices using smart devices or voice. But the problem is that they are not that cheap and moreover there can be privacy concerns regarding the data they collect. So, to address these problems we will make an inexpensive voice-controlled home automation system using easy to find components such as Arduino Nano, HC-05 Bluetooth module and relay driver board. The project utilizes an Android App that will convert our voice commands to text and then send it to the Arduino using Bluetooth. Depending on the command received the Arduino will control the connected appliances.
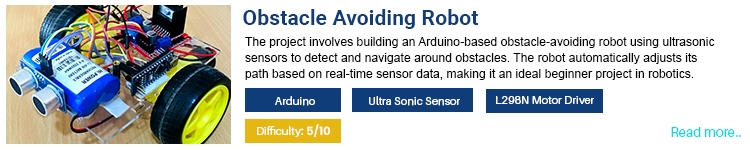
There are plenty of autonomous devices we come across in our daily lives, from autonomous vacuum cleaners to self-driving cars. One major feature of all these kinds of devices is that they are capable of detecting obstacles and objects around them and acting accordingly to avoid crashes or accidents. In this project, we will build such an obstacle-avoiding robot to learn the basics of how they work and how to improve them. Even though there are multiple methods to detect obstacles, we would be using an ultrasonic sensor-based approach they are cheap and easy to work with. In addition to the wheel driving DC motor, we are also using an additional Servo motor to change the ultrasonic sensor direction to make it easier to calculate the optimal driving manoeuvre.
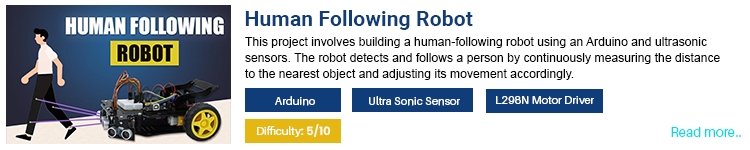
We have seen many types of autonomous robots including obstacle avoiding robots and line following robots. With this project we are going with a different approach and will build a human following robot. The basic working principle behind it is very simple and somewhat similar to an obstacle avoiding robot. But instead of changing direction when an object is detected, this robot will detect the movements and will follow the object. For detecting the object and movement we will use ultrasonic sensors. Unlike traditional designs, which use a single sensor and a servo motor to move the sensor around, we will use multiple sensors. These multiple sensors will be oriented at different angles. Using this method not only we can avoid the use of any moving parts for the sensor, but also the processing would be much faster since all of the sensors can be accessed at all times.
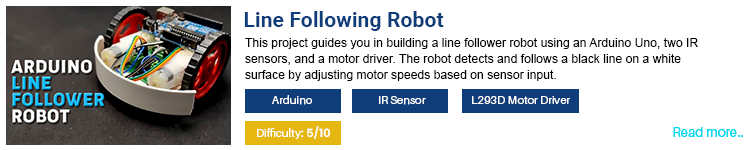
The line follower robot is a very interesting, easy to make autonomous robot. As the name suggests the line follower robot will follow the line drawn on any surface. To build this project we will use an Arduino UNO with a pair of IR sensors and a motor driver. The IR sensors will be placed in front of the robot on each side. The IR sensor contains an IR LED and an IR diode. The IR LED is illuminated always. The infrared light from the LED will be bounced back from any object that will reach the IR diode. Depending on the color of the object or surface the amount of infrared light reflected will be different. This difference is then used to identify the color of the surface. Using this we will determine if the robot crosses or moves away from the line. Since we are using two sensors, we can compare the reading from both sensors to determine to which direction the robot should be steered. Using this algorithm the robot will follow the line.
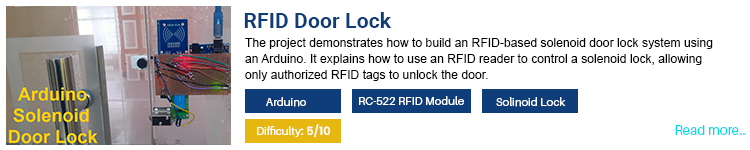
RFID tags are everywhere. From product labels to secure access cards. We have been using them for asset tracking, pet tracking, door locks for hotels and offices and many other applications. The advantages of an RFID system include the tags or cards don’t need any internal power source, they are fast, convenient and mostly secure. Why do we need to use a traditional key, which would take a fair amount of time and effort to lock and unlock, when the RFID door lock can do that within a fraction of a second by just placing the card near it? So, in this project, we will create such an RFID door lock using an Arduino UNO, an RC-522 RFID reader and a solenoid lock. A hall effect sensor is also used to detect whether the door is locked or not.
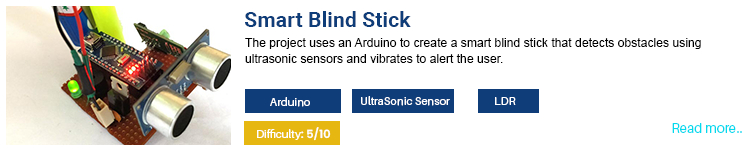
As we know it is hard for visually impaired people to navigate. They have to rely on their hearing and touch to navigate. But even with that, it would be harder to do so, especially in a busy street and other such places. We can help them overcome this difficulty using technology. For that, we will create a smart blind stick. This Smart stick will have an Ultrasonic sensor to sense distance from any obstacle, LDR to sense lighting conditions and an RF remote using which the blind man could remotely locate his stick. All the feedback will be given to the blind man in an audible form through a Buzzer. The buzzer can also be replaced with a vibration motor to get the haptic feedback.
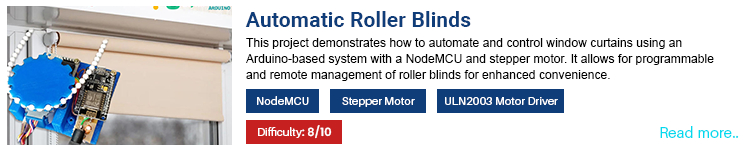
Nowadays most of our household devices are smart devices. how about automating our devices, so that they will operate automatically at a predefined time? You might remember the opening scene of the ironman Movie where the JARVIS AI opens up the curtains and greets Tony Stark. So, in this project, we are going to build an automated motorized blind controller. The main benefit is that this can be used with our existing roller blinds, and we don’t need to buy any special blinds for this project. To get started we have designed a few 3D printed parts, which include a window blind gear and a motor mount. The brain of this project is a NodeMCU development board. A tiny stepper motor is controlled using the NdeMCU at a certain interval to open and close the blinds. One other intriguing feature is that it can also be controlled using voice commands using IFTTT.
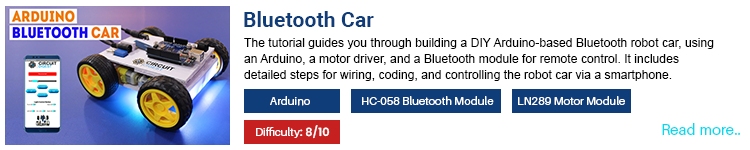
When we were kids, most of us would have been intrigued by remote-controlled toys. Even if we didn’t understand how they worked we were always eager to know the secret and make our own version of that. In this project, we will make such a remote-controlled toy: a Bluetooth controlled car to be exact. The project is fairly simple to make, and all the components are cheap and easy to find. The brain of the project is an Arduino UNO development board and for Bluetooth connectivity, we have used the HC-05 Bluetooth module. Four BO motors were used to drive the tyres and an L298N DC motor driver was used to control the motors. In addition to that we have also added a few programmable RGB LEDs to the circuit to make it a bit more visually appealing. The chassis and the body of the car are made from perf boards. The car can be fully controlled using a mobile app. Through the app, we can control the movement, speed and all the lights on the car.
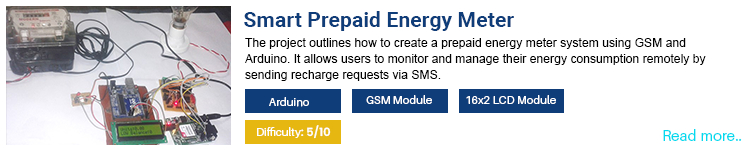
Have you ever thought about using electricity to recharge, just like our mobile phone? You might wonder why we need such a system when we already have a billing system. The main issue with the current billing system is that we don’t have any control over it. We don’t know if we are overusing it. But with this smart prepaid energy meter, we will have full control we will know when we are over a certain usage limit, and by knowing this we can monitor and control our energy usage as per our budget. One other advantage of this project is that we are using an exciting energy meter and interfacing it with an Arduino. By doing this we can ensure that the readings are accurate since the existing commercial meter is calibrated and certified but authorised agencies. The Arduino board interfaced with the analog energy meter using the calibration LED on the meter. The Arduino will count the calibration LED pulses to calculate the energy usage. A GSM module is also integrated with the Arduino to make remote recharge possible. Recharge can be done by sending an SMS. An LCD display is also provided to display the energy usage and remaining balance information.
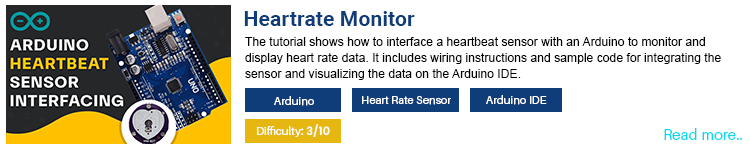
In This heartbeat monitor project, we will learn how to interface a heartbeat sensor with an Arduino board. The construction of the heartbeat sensor is fairly simple. The module contains an LED, an APDS9008 photodetector sensor, an opamp and a few complimentary components. When the LED is illuminated the light penetrates the skin and the blood vessels. The photodetector measures the amount of light that is absorbed or reflected back. As the blood vessels expand and contract with each heartbeat, the amount of light absorbed or reflected changes, allowing the sensor to calculate the heart rate. Using an Arduino, we will read this value and then display it in an OLED display.
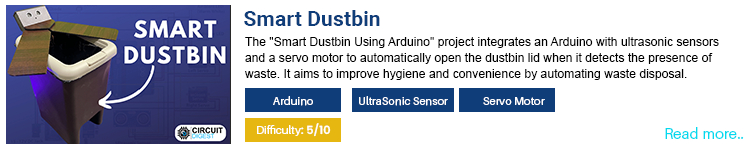
Properly classification and disposal of waste is a very important step in saving the environment. But one problem with using dustbins, especially the public ones is that people are always hesitant to open them because of are afraid that they might be contaminated or because disgusted to do so. Because of this people may even through the waste around without properly disposing it in a dustbin. To solve this issue, we have created our innovative smart dustbin. The smart dust bin is created using an Arduino Uno development board, an ultrasonic sensor and two servo motors. Whenever the ultrasonic sensor detects a movement within a certain set distance the Arduino will drive the servo motors, and the servo motors will open the dustbin cover that is directly attached to them. Once the waste is disposed into the dustbin and there is no movement the Arduino will then close the dustbin cover after a certain time. By using this smart dustbin, we don’t need to get our hands dirty every time we need to dispose of something into it.
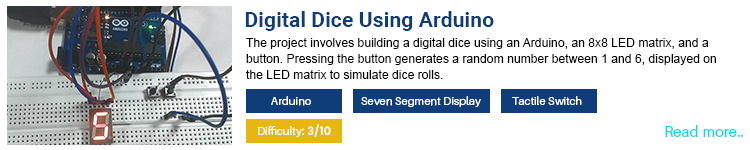
We all are familiar with classic board games such as the LUDO or the Snak and Ladder. We enjoyed playing them while we were kids. One thing common with these games is that we use dice to get a random value to play the games. One problem with traditional dice is that some may get paranoid that the other players can manipulate the dice by controlling how it is thrown. To avoid this, we use countermeasures such as a cup to shake the dice instead of using the hands. So, to avoid this why don’t we create something that can't be physically influenced? For that, we can create a digital dice, which will return a random number and display it on a seven-segment display. To build such a digital dice all we need is an Arduino development board, a seven-segment display, a pair of tactile switches and a few other passive components. When the button is pressed the Arduino will generate a random number and will display it on the seven segments.
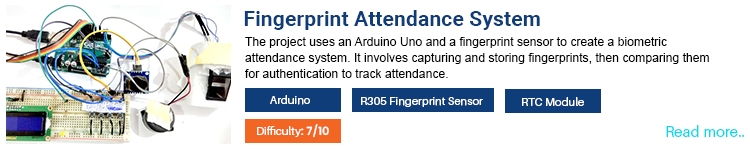
Attendance is very important in offices and educational institutions. There are many ways to mark attendance, from manual roll calling to advanced biometric or RFID-based systems. With this project, we will make a simple and easy to make attendance system using Arduino and a fingerprint sensor. For this, we are using an R305 fingerprint sensor. The R305 is an optical fingerprint sensor with the capability to store up to 512 fingerprint templates. The fingerprint sensor is interfaced with the Arduino using the UART protocol. The project also includes an LCD display for the user interface and a few tactile switches for user inputs. The project also includes an RTC module for accurate timekeeping.
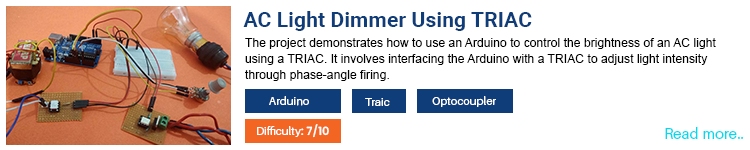
Controlling an AC appliance is very simple, all we need is a relay and some kind of microcontroller, right? It is simple if you only need to turn it on and off. What about a bit more control over them? For example, adjusting the brightness of a bulb or the speed of a fan. For that, we need another technique called phase angle control. So, in this project, we will learn about an AC lamp dimmer using Arduino and TRIAC. Here a TRIAC is used to switch the AC lamp, but you can also use this for any other inductive or resistive loads. We will also learn about zero crossing detection, its importance and how to implement it.
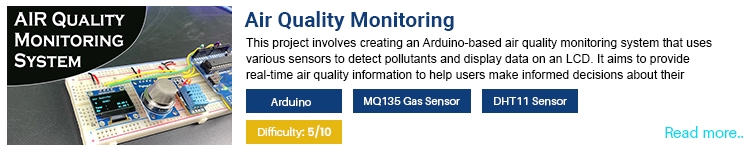
Air pollution is a major concern that adversely affects the environment, public health, and overall quality of life. It is a result of harmful substances being released into the atmosphere, including carbon monoxide, sulfur dioxide, nitrogen oxides, and volatile organic compounds. Air pollution around us can lead to respiratory diseases, cardiovascular problems, and other serious health conditions. This air quality monitor project is aimed at providing real-time insights into the air quality around us. This air quality monitor is built around an Arduino UNO featuring an MQ135 air quality sensor, a DHT11 temperature and humidity sensor and an OLED display to display all the information to the user.
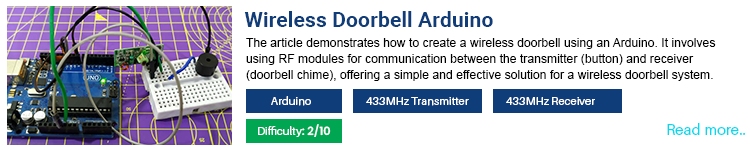
We are all familiar with wired doorbell systems. it is very essential for someone outside our door to call us. Even though they work, they have numerous disadvantages. Major advantages include they need to be installed by professionals to work properly, doorbell switches are normally connected to mains voltage and damages to the switch may result in electrocution and since the switch is normally situated outside humidity and water can also cause electrical leakage. Another issue with it is that it requires more work and time to install a wired doorbell system in an existing home. To overcome these issues, we are going to build a wireless doorbell. Since all of the parts are low-voltage components we can power them using batteries so that there is no risk involving the mains voltage. Not only that since it is wireless we don’t need to do any extensive wiring, and if powered with batteries they can act as a standalone unit without any wires or cables sticking out.
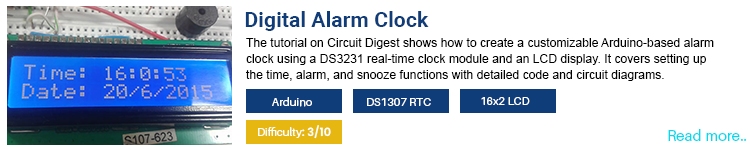
Don’t you get bored by ordinary analog alarm clocks? What about making your own alarm clock? That too with very minimal components. So, let’s build one our own. So, in this project, we are going to build a very simple and easy to make alarm clock. For this project, we are going to use an Arduino Pro mini with an RTC module, a buzzer and some other basic components. The DS1307 RTC chip will keep an accurate time even when the power is disconnected, all we need is to connect a small RTC coin cell to the RTC chip. The time will be displayed on a 16x2 LCD module, and the time and alarm can be set using a few tactile switches connected to the Arduino.
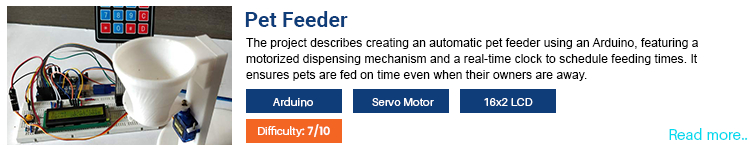
An automatic pet feeder is a modern solution designed to ensure pets receive timely and appropriate portions of food, even in the absence of their owners. Such an automatic pet feeder is particularly beneficial for busy pet owners who may not always be home to feed their pets at regular intervals. But the problem with the commercially available ones is that they are not particularly pocket friendly. So, we are going to build an easy to make, pocket friendly, DIY automatic pet feeder. With this DIY version, we can feed our pets in a set time. Our version of the pet feeder features an Arduino UNO as the brain, a 16x2 LCD for the user interface a matrix keypad for user inputs, an RTC module for timekeeping and a servo motor for controlling the dispensing mechanism. The dispensing mechanism is built using some 3D printed parts and can be integrated very easily.
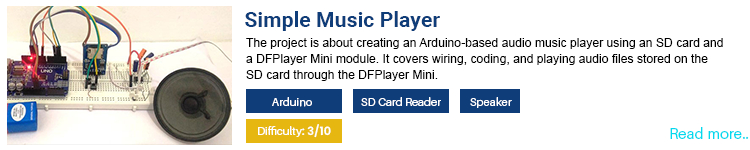
Adding sounds or music to our projects not only makes them look cool and attractive, but they would also come in handy to provide audible feedback to the user. In this project, we will learn how to do so, without the need for any special MP3 or music player modules. We will use a technique called pulse code modulation also known as PCM in short to generate the sound. The sound files will be saved in an SD card as a wave file and the Arduino will read them using an SD card module. The Arduino will output the sound through one of its GPIO and it will be amplified using an LM386 based audio amplifier before feeding to a loudspeaker. A couple of tactile buttons are also included in the circuit for the playback controls.
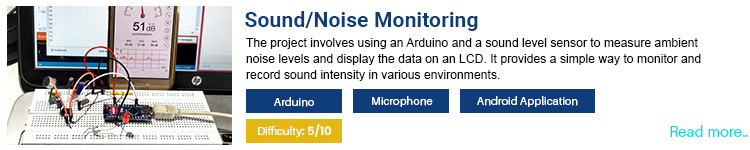
Just like any other type of pollution, sound pollution is also a major concern nowadays. Especially in cities where there is a lot of traffic and machineries that operates non-stop. Now you might wonder how exactly to measure sound pollution. For that, we have specialized equipment called decibel meters. which will give you an accurate reading of the noise or sound around you. So why don’t we make our own sound/ noise monitor to detect the accurate sound levels? For that, we are going to use a microphone attached to a preamplifier built around an LM386 amplifier chip. The output of the amplifier section will then be monitored using an analog pin of an Arduino board. The Arduino will then use this ADC reading to calculate the sound level in dB. The resulting value will then be displayed on a serial monitor for the user.
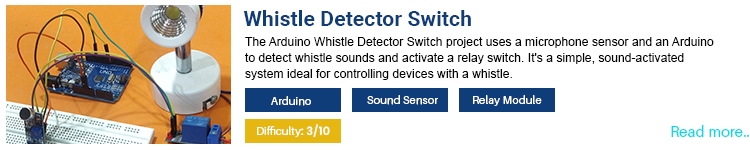
When we were a kid, most of us would have been fascinated with toys that were activated by claps or sounds. Not only just toys but there are even commercial as well as DIY versions of clap switches that can be used to control our lights or appliances with claps. Even though they work miraculously, they have one major disadvantage- they might get triggered by any kind of loud noises, like a loud radio or a neighbour’s lawn mower. So, to overcome this issue we are going to build a whistle detector switch. Unlike other sounds the whistle will have a uniform frequency for a particular duration and hence can be distinguished from speech or music. So we don’t need to worry about the false triggering. For this, we are going to use an Arduino Uno along with a sound sensor module, a relay and some other basic components. The sound sensor module will capture the sound using the onboard microphone and will give an amplified signal at the output. The frequency of this signal will then be calculated using an Arduino development board, and if the frequency matches that of a whistle the Arduino will activate or deactivate the relay resulting in controlling the load.
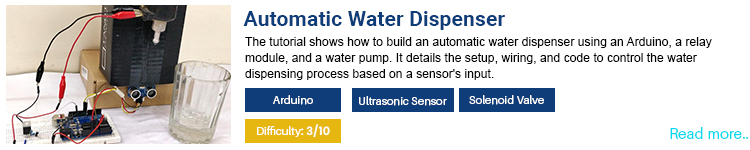
Even though our earth is mostly covered by water the amount of drinking water is very low. But the concerning reality is that this amount of drinking water is getting reduced drastically due to factors such as population explosion, pollution, climate change and water wastage. it is very important to avoid even a drop of water being wasted. It might not appear big at first time, but if your tap dripped a drop of water once every second it would take only about five hours for you to waste one gallon of water, that is enough water for an average human to survive for two days. So as a solution for such unwanted and unrecognised water wastage, we are going to build an automatic water dispenser which can replace the manual taps and reduce water wastage. Not only that as added bonus we can even avoid contamination from using the public taps. An Arduino Uno is used as the brain of this project. An ultrasonic sensor is used to detect the movement and if a movement is detected the Arduino will activate a solenoid valve to dispense a certain amount of water. After dispensing a set amount the valve will be automatically closed and thus avoid any water wastage.
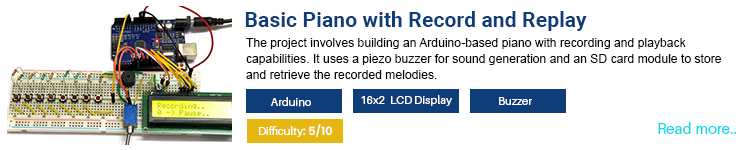
As a child, I was always fascinated by the music keyboard. It was always fascinating to see the musician not only playing new tunes but also combining them with prerecorded tunes. So why don’t we make a DIY version of that? So, in this project, we are going to build an electronic piano with the ability to record and replay the tones. An Arduino uno will handle all the processing and user inputs. An LCD display is provided for the user interface and a couple of buttons for user inputs. Depending on the button pressed the Arduino will save a corresponding tone to the memory. A simple piezoelectric buzzer is used to provide the sound output. For the button inputs, we have used a cleaver technique, that involves a resistor ladder network. by using this method, we were able to interface the whole 8 switches using a single analog pin.
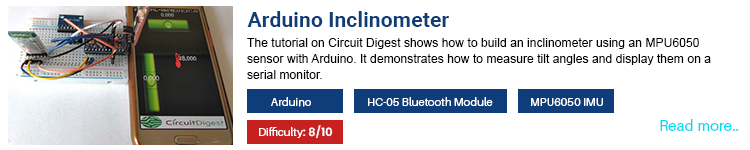
Inclinometers are used for measuring the angle of inclination of something or some surface. A simplified version of such an inclinometer is known as a spirit level. Traditionally, spirit levels have been the go-to tool in construction for ensuring surfaces are level and angles are accurate. However, they are limited to manual readings and lack digital precision. Even though there are digital inclinometers, they are expensive. So, we are going to build a digital inclinometer using Arduino. Our version of the DIY digital inclinometer consists of an Arduino Pro mini, An MPU6050 MPU and an HC-05 Bluetooth module. The Arduino will use the reading from the IMU to calculate the angles and the result will be sent to a smartphone via Bluetooth. Our custom-made Android app will display a virtual level with the readings corresponding to the reading from the Arduino.
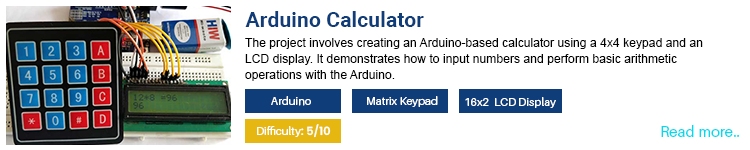
In the market, there are several types of calculators available, from simple arithmetic calculators to more advanced scientific and graphing calculators. In this project, we will learn how to make a simple calculator using an Arduino development board, an LCD display and a matrix keypad. The used inputs are captured using a 4x4 matrix keypad and the calculation result will be displayed on a 16x2 LCD display. With this project, we will learn how a matrix keypad works and how to interface it with an Arduino.
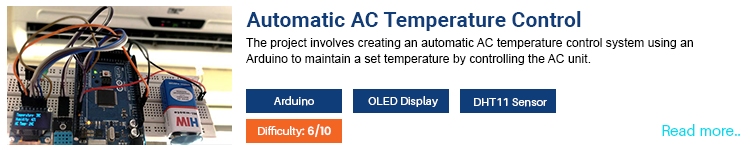
Air conditioners once considered a luxury have now become a necessity to combat the harsh climates. However, those who own an AC often share a common concern: high electricity consumption and the associated costs. This project aims to develop a compact Automatic Temperature Control Circuit designed to reduce electricity bills by automatically adjusting the AC's temperature based on the room's ambient temperature. By periodically varying the set temperature, we can prevent the AC from running at lower temperature settings for extended periods, thereby reducing power consumption and improving user comfort. The automatic AC temperature controller is built around an Arduino mega development board. A DHT11 sensor is used to detect the ambient temperature and humidity. A TSOP1738 IR sensor is used to decode the AC remote signal, and an IR LED is used to transmit the control signals to the AC unit.
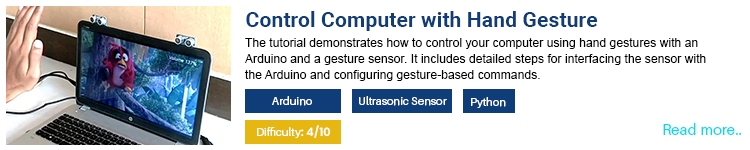
There are multiple ways to control a computer, using a keyboard, mouse or even with specialized remotes. How about hand gestures? Of course, we can use hand gestures. It would be interesting to make a device that can be used to control our computer with simple hand gestures. So, let's build one. For that, we are going to use a very simple setup consisting of only an Arduino UNO and a pair of ultrasonic sensors. We will place two Ultrasonic sensors on top of our monitor and will read the distance between the monitor and our hand using Arduino, based on this value of distance we will perform certain actions. For the PC side, we would utilize Python with pyautogui library. The best use case for such control would be the media control. We can simply control the media playback or volume controls using this setup.
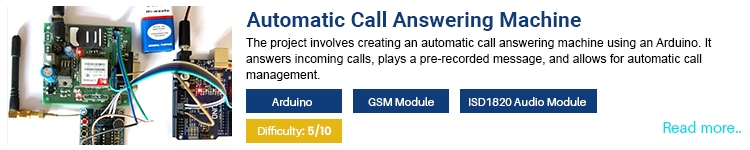
Most of the time when we call a customer support line, we are greeted with an Interactive Voice Response System also known as IVRS in short. Have you ever wondered how these IVRS systems work without any human intervention on the server side? To demonstrate it’s working we are going to build an automatic call answering machine. For the project, we are going to use an Arduino Uno development board as the main controller. A GSM module featuring SIM900 GSM SoC is used for the communication side of the project. The GSM module is interfaced with the Arduino using UART protocol and AT commands are used to control the module. For the audio section, we are using the ISD1820 Voice module. The ISD1820 module is capable of recording an audio clip for 10 seconds and then playing it when required. Whenever a call is received on the GSM module the Arduino will automatically answer the call. Once the call is answered the Arduino will start the audio clip playback. The audio output is connected to the mic input of the GSM module, thus whenever the audio is played the caller will be able to hear it.
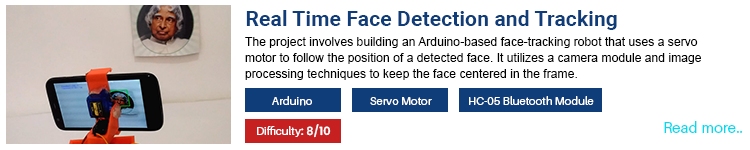
Building a real-time face detection and tracking system is not a simple task; it would require deep knowledge of programming and machine learning. But we can make a simple version of it without needing complex programming frameworks. To demonstrate that we are going to create a face-tracking robotic arm using an Android phone's camera and Arduino-controlled servos. The project uses Bluetooth to wirelessly connect the Arduino Nano to the Android phone, which handles image detection and sends movement commands to the servos. This setup includes SG90 servo motors and a 9V battery, allowing the robot to follow your face and keep it centred on the screen. The Android application, developed using Processing for Android, can be customized or downloaded as an APK file.
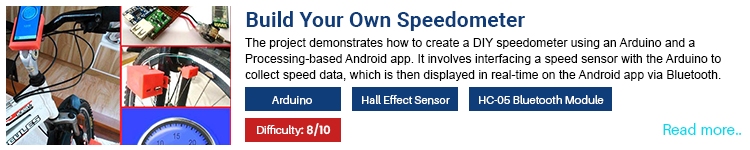
In this DIY Speedometer project, you can create a portable speedometer for bikes or vehicles using Arduino and a Processing Android app. Powered by an 18650 Lithium cell, the device is highly portable and can also charge your mobile phone while displaying speed. Speed measurement is achieved using a Hall sensor and a small magnet attached to the vehicle's wheel, with the Arduino calculating the speed based on the time taken for wheel rotations. The Arduino broadcasts speed data via Bluetooth to an Android phone. Our custom build application will display the received data on the phone using an attractive dial gauge. The application is developed using processing for Android and can be customized or downloaded as an APK file. The project guides you from start to finish, including mounting the kit on your vehicle.
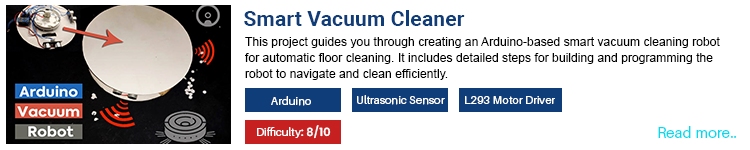
Robot vacuum cleaners have become increasingly popular due to their convenience and efficiency in maintaining clean floors with minimal human intervention. These devices can navigate around furniture, avoid obstacles, and cover large areas systematically, ensuring thorough cleaning. The problem with most commercially available ones is that they are relatively expensive. So building your own Arduino-based Smart Vacuum Cleaner Robot for automatic floor cleaning is an innovative solution for maintaining a clean home without the high cost of commercial products. This DIY vacuum cleaner robot is designed to be compact and practical, featuring ultrasonic sensors and an IR proximity sensor to navigate and clean efficiently. The ultrasonic sensors help the robot avoid obstacles, while the proximity sensor prevents it from falling downstairs. Powered by a 7.4V battery, the robot uses a modified portable vacuum cleaner motor for suction. The housing for the smart vacuum cleaner is built using easily available MDF boards.
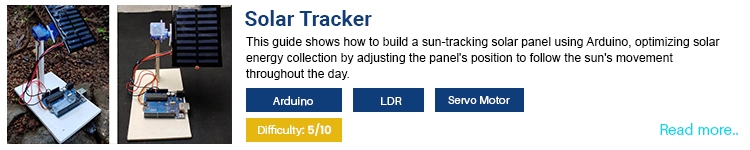
The best way to power our devices without destroying our earth would be to use renewable energy sources such as solar or wind power. But even though they are clean, pollution-free and don't need any fuel, they are highly dependent on some environmental factors. For example, wind turbines depend on wind speed and availability while solar panel depends on the availability of sunlight. To get the best performance from a solar panel all day long, it is necessary to align the solar panel in a way that it will receive the maximum sunlight throughout the day. For this, we would need a solar tracker. So, in this project, we would make an inexpensive but effective solar tracker prototype using an Arduino. two LDRs are used to detect the light intensity. Depending on the reading from the LDR the Arduino will re-align the solar panel using a servo motor.
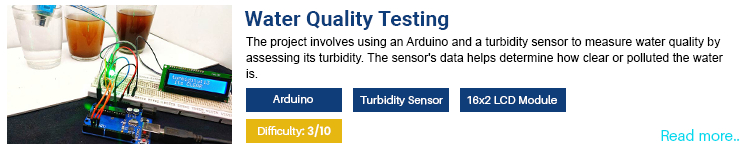
When it comes to water quality, one of the main testing standards is turbidity, which refers to the cloudiness or haziness of water caused by suspended particles, such as silt, clay, microorganisms, and organic and inorganic matter. It is a measure of how much light is scattered by these particles when shined through the water. A turbidity sensor would have two parts, a transmitter and a Receiver. The transmitter consists of a light source typically an LED and a driver circuit. In the receiver end, there is a light detector like a photodiode or an LDR. When a solution under test is placed in between the transmitter and receiver, the sensor would give an output signal depending on the solution. This project aims to build a turbidity meter based on such a sensor and an Arduino development board. The sensor is interfaced with the Arduino using an analog pin. The result will be displayed on a 16x2 LCD display.
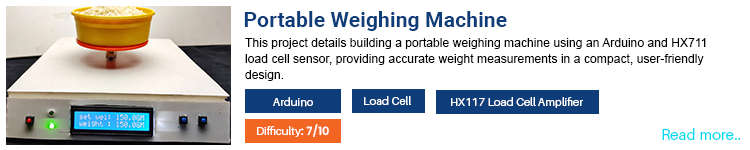
Have you ever wondered how exactly a digital weighing machine works? How do they give very precise weight readings? The modern digital weighing scales use a sensor called load cells. A load cell works by converting a mechanical force into an electrical signal using strain gauges. When force is applied to the load cell, it deforms slightly, causing a change in the electrical resistance of the strain gauges, which is then measured and converted into a corresponding electrical signal proportional to the force. this signal is then used to calculate the actual weight. To understand the actual working of a digital weighing machine, we are going to build a DIY portable weighing machine using an Arduino UNO board and a load cell with a load cell amplifier. The load cell amplifier module consists of HX711 IC, which is an amplifier chip specifically designed for this application. A 16x2 display is used for displaying the weight information to the user.
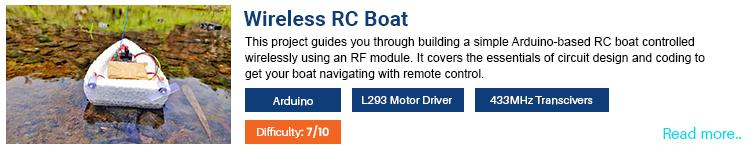
In this project, we will build a remote-controlled Arduino Airboat that can be controlled wirelessly using radio frequency. In the transmitter section, An Arduino Pro mini is used as the main controller. And for communication, we have used a 433MHz transmitter, that uses Amplitude Shift Keying (ASK) protocol. Tactile switches are used for user inputs. In between the Arduino and the transmitter an encoder chip is used. On the receiver side, the 433MHz receiver is connected to a decoder chip and the output of the decoder is then used to drive the motor driver. The entire body of the board is built using thermocol because it is lightweight and easy to handle with. Propellers are made using plastic sheets. The propellers are then attached firmly to the motor shafts and the motors themselves self are then attached to the boat’s body.